This article first appeared on the Institute for Computational Engineering and Sciences website.
The microwave oven has brought the same conveniences to chemists that it has brought to cooks, increasing the rate of chemical reactions and using less energy compared to traditional convection heating methods.
Some microwave chemistry experiments, however, yield results that are very different from those obtained by convection heating. These ‘specific microwave effects’ are often unexpected, unwanted, and difficult to explain, and include selective and superheating of materials. In an interdisciplinary collaboration, researchers at The University of Texas at Austin’s Institute for Computational Engineering and Sciences (ICES) teamed up with material scientists to understand, explain, and exploit a specific microwave effect. The team’s results were recently published in Scientific Reports.
First Attempts & Mysterious Thin-Film Growth
In early 2011, researchers at UT’s Texas Materials Institute and the Department of Mechanical Engineering were attempting to use microwaves to make thin films of semiconductor oxides. Such films are needed for a large variety of electronics, from solar cells to photodetectors. But growing them in conventional furnaces is a slow, high energy and high temperature process that restricts growth substrates to materials that can take the heat, like glass and metal. Flexible and light-weight substrates would melt away by the time the films could be grown in conventional furnaces.
However, If successful, microwave chemistry could accelerate the thin-film growth process, make it more energy efficient, and enable temperature-sensitive materials, such as plastics, to be used as growth substrates. This chemistry advancement could potentially enable the creation of plastic-based electronics.
Initial experiments with titanium oxide, performed in mechanical engineering professor Arumugam Manthiram’s lab by then graduate students B. Reeja Jayan and Katharine Harrison, were promising: Instead of two hours of baking at 450oC in a furnace, the researchers were able to make thin films in 15 minutes to one hour at temperatures as low as 150oC—lower than the norm for baking cookies. The process included coating a glass or PET plastic substrate with a very thin (about 150 nanometers thick) conductor layer, placing it in a solution containing titanium, and heating the solution in a microwave oven.
The experimenters were puzzled, however, to find that the titanium-oxide film clumped up at the edges of the coating used to promote the growth, leaving the center of the substrate nearly uncovered and unsuitable for use.
It was at this point that the material scientists approached Ali Yilmaz, an ICES core faculty member and an associate professor in the Department of Electrical and Computer Engineering. Yilmaz’s research group had recently made headlines with the advances they made in modeling and computation of electromagnetic power absorption by human tissues with an anatomical model called AustinMan. The experimenters were hoping that similar simulations could help inform their procedure and make the titanium oxide grow into uniform thin films.
Modeling, Computation, and Virtual Experiments
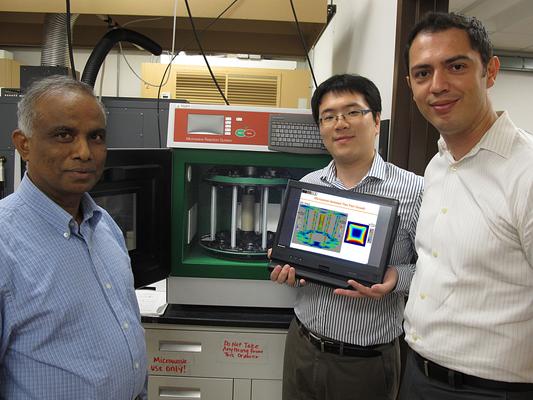
Arumugam Manthiram and researchers in his lab performed the microwave chemistry experiments while Kai Yang and Ali Yilmaz attempted to predict the outcome with computational simulations (named left to right).
Over the next year, Yilmaz and Kai Yang, an electrical and computer engineering graduate student, modeled the main components of the experimental setup and developed formulas, algorithms, and software to analyze the problem.
“There was a lot of detective work to figure out the critical parts of the experiment that had to be modeled. Of course, all of us wanted to have the simplest model possible and get answers immediately, but we quickly found out that simple models failed to agree with the experiments,” Yilmaz said.
This led the computational engineers to gradually increase the complexity of their models as they kept comparing the results of the simulations with the experiments. Eventually, they were able to perform simulations whose results not only agreed with the observations in the lab but also could predict the outcome of new experiments.
“Out of necessity, the final model we developed turned out to be very detailed,” Yang said, “in addition to the coated substrate and the solution containing the titanium, we had to model the quartz vessel holding the solution, the metallic rotor holding the vessel, the movement of the rotor, and the microwave oven.”
As the complexity of the model increased, however, computations became increasingly more challenging.
“Simulations became impossible with classical computational methods as the complexity of the model increased” Yang said.
The researchers developed an advanced algorithm, parallelized it so different parts could be computed simultaneously, and used hundreds of processors on the supercomputer Lonestar at the Texas Advanced Computing Center to be able to solve the equations governing the field distribution in their model. In a forthcoming paper, Yang and Yilmaz show that on Lonestar they can perform these simulations, which would take an estimated ten years with a classical method on a modern desktop computer, in less than one day and still obtain sufficiently accurate results.
The simulations showed that the electric field was congregating at the edges of the thin conductive layer, depositing much more energy to its edges than its center. This clued the researchers in that the conductivity of the coating was a key factor; so they started performing virtual experiments to explore what modifying the conductivity would do.
They found that they could deposit the energy much more uniformly—and as a result grow more uniform films—if they lowered the conductivity of the thin coating layer by a factor of 100 instead of increasing it as the material scientists initially expected. Despite their doubts, the material scientists reduced the conductivity of the coating layer, repeated the experiment, and found out that they were able to obtain the more uniform thin films that they were after from the start. The dramatic results helped the researchers establish the role of the conductive layer in the outcome with a lot fewer experiments than usual. Instead, a host of virtual experiments and a few physical ones proved that there is an optimal range of conductivity values for obtaining uniform films.
“We could do the [conductivity] experiments in just two trials based on what the model predicted, as opposed to say another 50–100 experiments we would normally do,” Jayan said.
Explaining Microwave ‘Magic’
While the ultimate goal of the simulations was to find a way to improve the film uniformity, the researchers were also trying to confirm the presence of specific microwave effect.
“[Specific microwave effects] are kind of like a magic that happens only if you use a microwave” Jayan said. “It is very difficult to prove what is happening.”
When Jayan presented their initial experimental results at a conference, skeptical audience members attributed them to simply rapid heating rather than a specific microwave effect and recommended re-running the experiments in a furnace. The film did not grow at all in this case.
“What we effectively found is that if you repeat our experiments and replace the microwaves [with another heat source] they won’t work,” Jayan said.
And while they suspected that the thin coating layer had something to do with the specific microwave effect, they didn’t know why this was the case or how they could control the effect. Once again, the simulations turned out to be valuable.
“One of the biggest advantages of computational science and engineering is that you can observe quantities that are not accessible in experiments. In the simulations, we were able to observe where the microwave energy was deposited—an input of the reaction—whereas in the experiments we could only see the film growth—an output of the reaction,” Yilmaz said.
The simulations showed why the reaction could only occur in the microwave oven: The “selective heating” of the thin coating layer by the microwave fields made the coating absorb almost as much energy as the titanium-containing solution that organized into the film. This special selectivity of the microwaves between the different materials is a specific microwave effect that can’t be created by convection heating.
“Specific microwave effects can be controversial because it is difficult to explain exactly how they work,” Yilmaz said. “Once we started doing our simulations, the experimental results started to make sense. We showed that the film growth was not an anomaly, that this is actual physics in the microwave oven, and that we can exploit it to engineer materials in new ways. It was a big accomplishment for predictive computing.”
More Films, Substrates, and Simulations
The results with titanium oxide films are encouraging; the experimental films were tested in photo-detectors, hybrid solar cells, and lithium ion batteries with the devices functioning in each case. The researchers are also investigating whether the selective heating effect can be exploited to grow other materials into different shapes and sizes of thin films and on different substrates.
The research will likely influence industrial semiconductor oxide film growth and may enable a whole new set of low-temperature growth substrates, from plastics to textiles.
“Our experimental demonstration to grow highly crystalline thin films of titanium oxide at such low temperatures with microwave-assisted solvothermal processes opens up a new arena,” Manthiram said.
It could also impact how microwave chemistry is performed in the future as predictions from virtual experiments supplement the ones in the lab. The U.S. Army Research Lab has already expressed interest in using similar computational models to improve the microwave-assisted growth of ferroelectric materials.
“Some materials will be very difficult to grow experimentally, but if the model can predict the possibilities then the experiment will have at least a chance of getting some success,” Jayan said. “So going forward, the model and its predictive nature are going to be hugely important.”